Gravitational lensing is the main scientific tool of our project: this effect is the deflection of light coming from background objects due to the distortion induced in the space-time metric by objects, thus acting as “lenses”, located between the observer and the source.
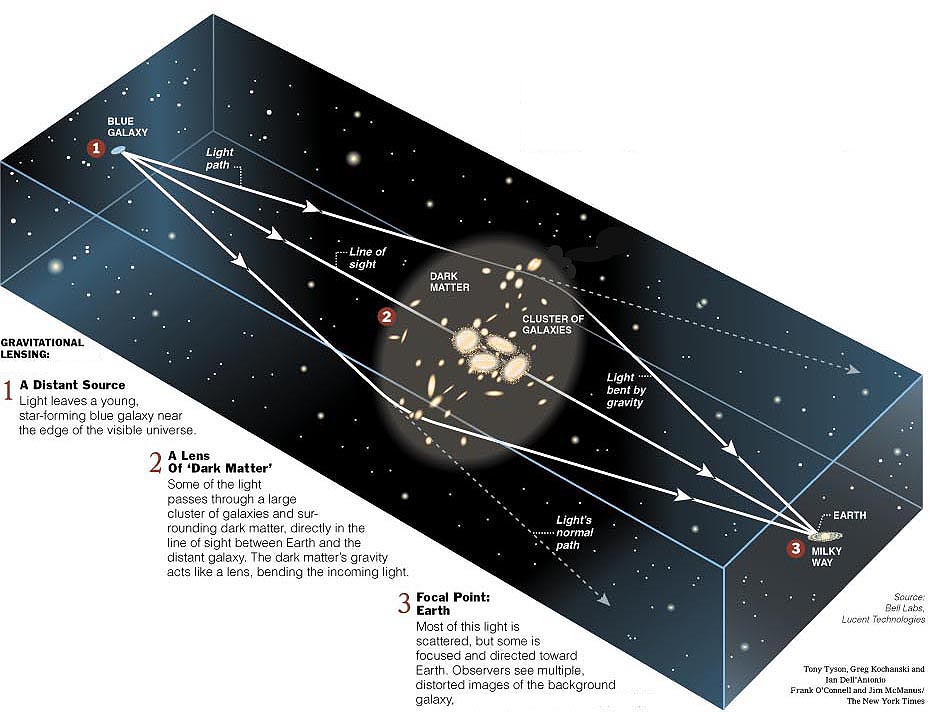
Image credit: Bell Labs / Lucent Technologies, plus Tony Tyson, Kochanski, Dell’Antonio et al. / NY Times.
For a visualisation of the lensing effect in action, you could have a look at a nice animation provided by NASA/ESA: you can find it below or following this link.
Credits: NASA, ESA & L. Calçada
The lensing effect can give us access to the mass content of clusters and is the most direct probe of the dark matter content, allowing to precisely determine the total mass distribution in galaxy clusters, without any assumption on the type of the intervening matter. One can distinguish several regimes in gravitation lensing, as illustrated below.
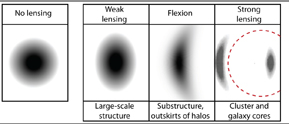
Image credit: ESA/Euclid
Strong lensing events occur when multiple, distorted images of a background source are produced by a close alignment with a foreground intervening massive lens along the line of sight.
In the weak lensing regime, the distortions induced on the background image are smaller, and no multiple images are produced.
In the weak lensing regime, one can distinguish between second-order (shear) and third-order (flexion) signal. The higher-order signal, flexion, is sensitive to small-scale variations in the lensing field, and therefore is a promising probe for the mass distribution at small, galactic scales.
Most of these probes require a statistical treatment of a large sample of clusters in order to beat down noise and to learn about sample variance. We have therefore assembled a sample of nearly 80 clusters, observed with multiband wide field imaging Megacam and SuprimeCam facilities mounted at the CFHT and Subaru telescopes in Hawaii, respectively.
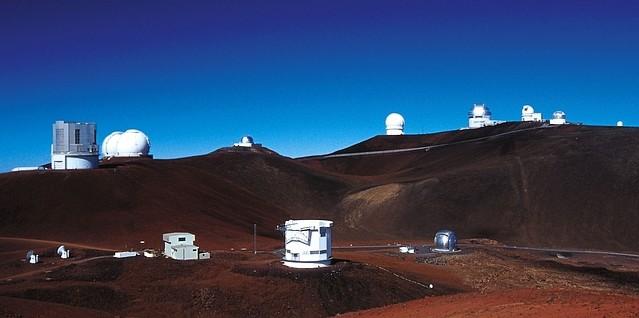
The primary goal of the Amalgam project is the study of the mass distribution of a large sample of clusters of galaxies, which are known to contain large amounts of Dark Matter and constitute the largest condensed objects in the Universe. It is crucial to improve and assess the current methods to estimate the mass of galaxy clusters, as most of the methods based on galaxy clusters as cosmological tools require an accurate determination of their mass.
Besides this main scientific goal, we are interested in tackling several other important issues in galaxy cluster astrophysics, such as:
Besides the Dark Matter content of galaxy clusters, smaller amounts of baryons are responsible for most of the information one can access with ground- or space-based observatories:
- the hot intra-cluster gas (or Intra-cluster medium, hereafter ICM) at temperatures of a few keV emitting in X-rays;
- the stellar component, including both the stellar content of cluster galaxies and the diffuse stellar population bound to the overall cluster potential (the latter constituting the Intra-Cluster Light, ICL);
- the warm, ionized gas that could be present in the core of galaxy clusters.
Several fundamental questions regarding the cluster baryonic components still need to be definitely assessed: for example, the formation mechanism of the Brightest Cluster Galaxy (BCG) has not been conclusively determined, despite several hypotheses triggered both from numerical and from observational findings. Similarly, a definitive picture of the formation of the diffuse stellar component found in the halos of cD galaxies and of the ICL has not been reached yet. Moreover, it is still to be assessed the effect of the evolution of the baryonic components on the local distribution of dark matter.
Therefore, we aim at further investigating the mechanisms regulating the evolution and interplay of the baryonic components in galaxy clusters.
N-body simulations of hierarchical structure formation in CDM models show that the density profile of simulated dark matter halos, for a wide range of masses, can be described through a simple analytical function, depending only upon two parameters – the halo concentration and scale radius. Moreover, the halo concentration and mass are expected to be correlated: halos having larger masses should be less concentrated, with the expected concentration being dependent upon the assembly redshift, and thus on the adopted cosmological model.
Therefore, an important test to the LCDM scenario is the comparison of the concentration/mass relation predicted from numerical simulations with the observational results.
Through the lensing analysis of our extended cluster sample, we aim to estimate the observed concentration-mass relation from massive clusters in an extended redshift range.
It is well established that galaxy populations in clusters differ from field galaxy populations. Unlike field galaxies, the evolution of galaxies in clusters, is influenced by severalenvironmental mechanisms (such as ram pressure stripping or galaxy harassment) that can affect their overall properties. In particular, the mass distribution of early-type cluster galaxies is expected to be truncated with respect to that of comparable field galaxies, due to the tidal stripping of the galactic halos by the global cluster potential.
However, these environmental effects on cluster galaxy evolution are not completely understood not assessed, and many aspects still have to be clarified.
It is clear that determining the distribution of dark matter is a key piece of the puzzle. To this goal, we are going to systematically measure the shape and density profile of cluster members, taking advantage of the lensing information both in the weak (shear+ flexion) and strong regime. We will thus derive robust constraints on the effect of tidal interaction on cluster galaxy halos on small ( strong lensing), intermediate ( flexion) and large ( shear) scales.
The mass of galaxy clusters is directly measurable thanks to the lensing effect; however, deriving lensing mass measurements is observationally expensive, especially for cosmological applications of galaxy clusters, that require statistically significant cluster samples. Therefore, a widely used approach is to rely on
scaling relations that connect the total cluster mass to physical quantities which are easier to observe, for example the X-ray temperature. These scaling relations are reflect the formation and evolution of clusters, so they can provide information on the physical processes at play; and since many studies based on galaxy clusters as cosmological probes take advantage of mass-observable scaling relations, it is important to calibrate them in a robust way. For these reasons, once the analysis of our cluster sample is completed, we aim to compare the lensing masses with the observables that can be obtained, e.g., from X-ray data.
Our progress and results related to the mass profile of galaxy clusters are developed here, while those on mass properties of cluster galaxies seen as substructure in the overall cluster mass distribution are listed here.
All the difficult tasks of galaxy shape measurements, the detection of lensing signal, the distinction between foreground and background galaxies as well as star/galaxy separation are tackled in this project. This page will regularly show technical progresses and achievements.
Cover image: the Bullet Cluster (1E 0657-56)
Composite Credit: X-ray: NASA/CXC/CfA/ M.Markevitch et al.;
Lensing Map: NASA/STScI; ESO WFI; Magellan/U.Arizona/ D.Clowe et al.;
Optical: NASA/STScI; Magellan/U.Arizona/D.Clowe et al.